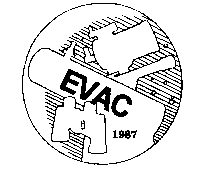
EAST VALLEY
ASTRONOMY CLUB
VIRTUAL BEGINNER'S LAB
How Do I Get Started In Astronomy?
The fact that you are reading this means that you are started in the right direction. The natural tendency is to purchase a telescope and then try to figure out how to use it. However, the best way to begin your personal exploration into astronomy is by reading! Visit your local library and look for books on astronomical topics, geared to a novice level. Bookstores are another great source of reading material - the larger stores will even permit you to peruse the literature over a cup of coffee.
The Internet is another great source of information. Use your favorite search engine and enter keywords such as 'amateur astronomy'.
Seek out other amateur astronomers. Joining an astronomy club is one of the smartest things a newcomer to the hobby can do. And, with annual dues of only $30, East Valley Astronomy Club, Inc. (EVAC) is a great choice!
Above all, resist the urge to buy a telescope for now. There's much to be learned before making that purchase.
Beginner Telescopes
Read the article All About Beginners' Telescopes by Phil Harrington, Astronomy Magazine contributing editor and author of numerous astronomy books, including the best-selling StarWare. Also, there is an interesting article by Brian Taylor, Reflecting vs. Refracting Telescopes: 7 Key Differences.
Recommended Books
True, the choices seem endless. Here is a short list of some highly regarded introductory books.
-
StarWare (3rd Edition) by Philip S. Harrington
-
Night Watch by Terence Dickinson
-
The Backyard Astronomers Guide by Terence Dickinson and Alan Dyer
-
Turn Left at Orion by Guy Consolmagno and Dan M. Davis
-
The Stars - A New Way to See Them by H. A. Rey
-
The Year-Round Messier Marathon Field Guide by Harvard Pennington
-
Bright Star Atlas 2000.0 by Wil Tirion and Brian Skiff
-
365 Starry Nights by Chet Raymo
-
Touring the Universe Through Binoculars by Philip S. Harrington
-
Choosing and Using a Schmidt-Cassegrain Telescope by Rod Mollise
-
Astronomy magazine
-
Sky & Telescope magazine
This is by no means a complete list, only a starting point.
Why Learn The Sky?
It is not necessary to learn the sky, but it is highly recommended! As your knowledge grows, your enjoyment will be enhanced by knowing the relative positioning of objects in the sky.
Start slowly by familiarizing yourself with the constellations. Head outside on a clear night and gaze skyward. No equipment is necessary for this part of your journey.
Using a book for reference, try to identify as many constellations as you can. Take special note of the positioning of the constellations with regard to time and date. With practice the constellations will soon become as familiar as old friends.
Depending on your level of local light-pollution, binoculars may be helpful to see the primary stars in each constellation.
Why Learn The Constellations?
Realize that a good understanding of the constellations will make it that much easier to use various charts, and to learn new skills such as star hopping. So, proceed at your own pace.
The only necessary equipment are your eyes and a star chart. But, the whole process is much nicer if you add the following: a planisphere, a spiral notebook, and a flashlight with a red lens (preferably red LED). Binoculars would make this list complete.
Begin the process slowly, maybe concentrating on two or three constellations in an evening. Start with the brighter constellations, such as Orion, Ursa Major, Cassiopeia or Gemini. Can you see all of the primary stars naked-eye? Sketch or take notes as you proceed... whatever facilitates the process of learning.
Of course, you simply cannot learn all of the constellations quickly. Many of them are only visible during particular seasons. Some are in the southern hemisphere and are never visible here. You should plan on taking about a year to work your way through the list of constellations.
As you progress through this adventure, take note of the way in which the constellations move across the sky. Those constellations near the North Star (Polaris) are visible throughout the year. They are referred to as circumpolar. Cepheus, Ursa Major and Cassiopeia are circumpolar constellations.
Some constellations, Cygnus, Leo and Hercules, for example, rise in the east and set in the west. They are visible about half of the year. Another group of constellations rise in the south /southeast and set in the south / southwest, and are only visible about a third of the year. They are always low in the sky, and therefore, may be hard to view from your location. This group includes Cetus, Hydra and Scorpius.
What Is Chromatic Aberration?
Chromatic aberration is associated with the objective lenses of refractor telescopes. It is the failure of a lens to bring light of different wavelengths (colors) to a common focus. This results in a faint violet halo around bright stars, the planets and the moon. It also causes a reduction in contrast. This defect is more prominent as speed and aperture increase. Achromat doublets reduce this defect. Sophisticated and expensive designs using more exotic glass, can virtually eliminate the defect altogether.
Power / Magnification Explained
First of all, magnification is one of the least important factors in the telescope purchase decision!
Magnification is actually the product of the relationship between the telescope itself and the eyepiece being used. To determine the power, divide the focal length of the telescope (in mm) by the focal length of the eyepiece (also in mm). By exchanging eyepieces of varying focal lengths in the same telescope, you can increase or decrease the magnification accordingly.
For example: an 8" Celestron SCT has a focal length of 2,032 mm. When a 30 mm eyepiece is used, the magnification is 68x (2032 ÷ 30 = 67.73). If I then use an 8 mm eyepiece, the power becomes 254x (2032 ÷ 8 = 254).
Since eyepieces are interchangeable, the same telescope can be used at different powers for different applications. But, like in everything else, there are practical limitations regarding the upper and lower limits of power. The laws of physics, as well as the physiology of the human eye, introduce practical limitations. A good rule of thumb is that the maximum usable power is equal to about 60 times the aperture of the telescope (in inches), under ideal conditions.
So, using our preceding example of the Celestron 8" SCT - the rule tells us to expect about 480x as the maximum power. Visible detail and sharpness both decrease as power increases.
Aperture Explained
Aperture is the single most important factor in selecting a telescope. Since the primary function of a telescope is to collect light, the larger the aperture the better the image produced.
The aperture of a telescope is the diameter of the objective lens (refractor) or primary mirror (reflector or catadioptric) specified in either inches, millimeters or centimeters. The larger the aperture, the more light is collected. The more light collected, the brighter the image. Greater detail is apparent as aperture increases.
Carefully examine your budget and portability requirements to enable you to select a telescope with the largest possible aperture.
What Is Focal Length?
The focal length is the distance (in mm) from the lens or primary mirror to the point in the optical plane where the telescope reaches focus (the focus point). The longer the focal length of a telescope, the larger the image and the smaller the field of view. Focal length is the aperture (in mm) times the focal ratio.
Using our Celestron 8" SCT example we can determine that the focal length is 2,032 mm. This is calculated by multiplying the aperture (8" or 203.2 mm) by the focal ratio (f/10). So, 203.2 x 10 = 2,032. The manufacturer usually specifies the focal ratio on the instrument.
What Is Focal Ratio?
The focal ratio is the ratio of the focal length of the telescope to its aperture. This is calculated by dividing the focal length (in mm) by the aperture (also in mm). Again, using our 8" SCT example: 2,032 mm focal length divided by 203.2 mm aperture equals 10. (2032 ÷ 203.2 = 10) This is usually specified as f/10.
Many people want to equate focal ratios with image brightness, but this is really only true when using the telescope photographically on 'extended' objects such as the Moon or a distant nebula. The brightness of a star, a point source of light, is entirely a function of aperture - the larger the aperture, the brighter the image. When viewing extended objects, the apparent brightness at the eyepiece is a function of aperture and magnification, and is not related to focal ratios. Extended objects will always appear brighter at lower magnifications.
Telescopes with small (called fast) focal ratios will produce brighter images of extended objects on film, thus requiring shorter exposure times. Generally speaking, the main advantage of having a fast focal ratio (when used visually) is that it will deliver a wider field of view.
-
Fast focal ratios are f/6 and lower.
-
Medium focal ratios are f/7 to f/11.
-
Slow focal ratios are f/12 and longer.
Limiting Magnitude
A system of magnitudes is utilized in astronomy to indicate the relative brightness of a stellar object. Objects are assigned numerical magnitudes. The larger the number, the fainter the object. Each successive magnitude number represents a decrease in brightness of about 2½ times. Generally speaking, the faintest star that can be seen with the unaided eye (no telescope) is about sixth magnitude.
The faintest star that can be seen with a telescope, under excellent seeing conditions, is defined as the limiting magnitude. As you can imagine, limiting magnitude is directly related to aperture. Limiting magnitude is often reduced by atmospheric conditions and the visual acuity of the observer. Photographic limiting magnitude is approximately two or more magnitudes fainter than visual limiting magnitude.
Seeing Conditions Explained
'Seeing' is the term astronomers use to describe the atmospheric conditions. The atmosphere is in constant motion - changing temperatures, air currents, weather fronts and particulates. If the stars appear to be twinkling, it can generally be inferred that the seeing is poor; conversely, when star images are steady the seeing is good.
Poor seeing is much more noticeable when observing planets and the Moon, whereas deep sky objects are less affected by poor seeing. With deep sky objects the most important factor is transparency (a measure of the sky's darkness as determined by clouds, dust, haze and light pollution). Seeing conditions and transparency vary widely from site to site, from season to season, and from night to night.
Field Of View
The amount of sky visible through the eyepiece is referred to as the true field of view, and is measured in degrees of arc (angular field). The larger the field of view, the larger the section of sky that is visible. Angular field is calculated by dividing the apparent field of view of a given eyepiece by its resultant magnification.
Manufacturers usually specify the apparent field of view (sometimes referred to as AFOV) in degrees for their eyepiece designs. The larger the apparent field of view of an eyepiece, the larger the true field of view (sometimes referred to a TFOV). Likewise, lower powers yield larger fields than higher powers.
Using our 8" SCT example with a 25 mm Antares plossl eyepiece, the eyepiece yields 81x, and its AFOV is 52° (as specified by the manufacturer). The TFOV is then 0.64° or about two-thirds of a degree.
-
Magnification: 2032 ÷ 25 = 81.28 or 81x
-
AFOV is provided by the manufacturer, or can be calculated in the field using star drift techniques.
-
TFOV: 52 ÷ 81.28 = 0.639763779 or 0.64°
What Is Collimation?
Collimation is simply the process of properly aligning the optical components of the telescope to ensure it is delivering optimal images. Collimation is critical for achieving optimum results. Poor collimation will result in optical aberrations and distorted images, and is usually to blame when a new reflector user has determined that his/her new telescope is 'junk'. Collimation is not only concerned with the alignment of the optical components, but also with their alignment with the mechanical tube.
-
Click right here to be transported to Mel Bartels' Dynamic Collimation page.
-
If you have a Schmidt-Cassegrain telescope (SCT) click here to download an overview of collimating your optics, written by Rod Mollise.
Zodiacal Light
Zodiacal light appears as a faint, slanted cone-shaped glow in the west after twilight or in the east before twilight. On clear moon less nights in Arizona it can be seen extending from the horizon to past the central meridian. Scattering of sunlight off dust particles causes this faint light in the earth's orbital plane. Zodiacal light is best seen in the evening during March and April and in early morning hours during September and October. If your eyes are well dark-adapted it can easily be seen with the unaided eyes during these times.
If you attempt to observe something located in this area it will not display the amount of detail you may expect because the zodiacal light reduces the contrast, which means you will not see the amount of detail expected. In the spring, if you are observing galaxies, this reduced contrast can be quite aggravating.
To measure the difference for yourself, try this experiment. Plan to observe something in, say April, just past twilight. Do not observe anything bright, perhaps a galaxy or a planetary nebula. Make sure it is in the zodiacal light for this observation; take careful notes. Make another observation at a different time when this same object is not in the zodiacal light. The duration between the two observations may be as much as a year, so take good notes for each observation.
Compare the two observations, drawings if you have them (you just knew I'd get that plug in). Draw, mentally that is, your own conclusions. It gets worse if a bright planet, like Venus, is embedded smack dab in the middle of this irritating observing interference.
What Is a Wedge?
An equatorial wedge is normally used with a fork-mounted telescope to tilt the instrument toward the celestial pole. This 'tilt' is an angle equal to your latitude, so that a clock drive functions properly and setting circles provide accurate readings.
The wedge is positioned between the tripod and the bottom of the fork mount.
Setting Circles
Setting circles are available in two types: manual and digital.
Either variety facilitates the process of locating celestial objects according to their coordinates, which are listed in star charts. For setting circles to function properly, the telescope must be equatorially mounted and polar aligned.
Digital setting circles utilize an optical encoder on each axis and a computer that contains an extensive database of objects and their coordinates.
Right Ascension and Declination
On the Earth the position of a particular location is measured using latitude and longitude. Latitude is measured by parallel lines that indicate the number of degrees north or south of the equator. The north pole is +90° and the south pole is -90°. Longitude is measured by lines extending between the poles, 180°E and 180°W of a zero-degree line running through Greenwich, England.
Astronomers employ a similar set of coordinates in the sky. The celestial equator is an imaginary line around the sky, positioned above the Earth's equator. The celestial poles are the imaginary points where extension of the Earth's axis into space would meet the celestial sphere.
In astronomy, the coordinate similar to longitude is called right ascension (RA). A line extending from the celestial north pole, through your zenith, to the celestial south pole, is called the meridian. The meridian crosses the celestial equator perpendicularly, forming a line of constant right ascension. RA is measured along the celestial equator. Because the sky appears to rotate once overhead every 24 hours, RA is measured in hours.
The celestial equator is divided into 24 hours of RA. Because the sky appears to turn a full circle (360°) each 24 hours, each hour of RA is equal to 15°, and each minute of RA is equal to 15' (minutes of arc, or arc minutes). Each second of RA is equal to 15" (seconds of arc, or arc seconds). While at first it may be confusing, remember that in this context 'minutes' and 'seconds' refer to units of arc, not time.
The celestial coordinate analogous to latitude is called declination (DEC). Declination is measured in degrees, minutes and seconds of arc north (+) or south (-) of the celestial equator. The celestial equator has a DEC of 0°, the celestial north pole is +90° and the celestial south pole is -90°.
The stars are essentially fixed in the sky, so their RA and DEC do not change over short periods of time. The Sun, Moon and Planets move through the sky with respect to the stars, so their RA and DEC changes over time. Right ascension is typically abbreviated as RA, r.a. or indicated by the Greek letter α (alpha). Declination is abbreviated as DEC, dec. or with the Greek letter δ (delta).
What is Conjunction?
The alignment of two celestial bodies occurring when they reach the same celestial longitude is called conjunction. The two bodies appear close to each other in the sky. During inferior conjunction a planet whose orbit is inside Earth's orbit passes between the Earth and Sun. In superior conjunction, a planet whose orbit is inside that of Earth passes on the far side of the Sun, with respect to the Earth.
Argument of Perihelion
Argument of Perihelion
This is a measurement used in defining the shape of an object’s orbit. It’s used in combination with other numbers, called orbital elements, which provide a complete definition of the orbit. The symbol used to represent Argument of Perihelion is usually the lower case Greek letter omega. The parameters that specify the position and motion of a body in orbit are called the orbital elements. Six parameters are required to uniquely define a body's orbit.
The Argument of Perihelion is defined as the angle (in the body's orbit plane) between the ascending node line and perihelion measured in the direction of the body's orbit.
Perihelion is defined as the distance between the orbiting body and the sun at it's closest approach.
The longitude of the ascending node is defined as the angle between the reference X-direction (typically the vernal equinox) and the point at which the body passes up (north) through the reference plane. This angle is often referred to as the upper case Greek letter omega.
The longitude of perihelion is defined as the sum of the longitude of the ascending node and the argument of perihelion.
Why Do I Need a Finder Scope?
Most beginners are surprised to learn how 'little' sky is observed through the eyepiece at moderate and high magnifications. This can make the process of locating objects quite difficult, if not impossible, without some assistance. Enter the finder scope.
Finders are available in two varieties: the traditional finder scope and something called a unit-power finder.
The traditional finder scope resembles a miniature refractor, and is usually mounted atop the main telescope near the eyepiece. They provide low magnification coupled with a wide field of view and crosshairs (reticle). Once aligned with the main instrument an object centered in the finder will also be centered in the main telescope's view.
The unit-power, or reflex, finder is a popular alternative to the traditional finder scope. As the name implies, the unit-power finder provides no magnification. This design, using LEDs, projects either a red dot or a series of circles onto a small piece of glass against the background of the night sky. Its operation is intuitive, but doesn't work as well in light-polluted areas because the user is limited to naked-eye objects.
Which Eyepieces To Purchase
Follow this link to an excellent resource on choosing the right eyepiece.
Which Telescope To Buy
Once you begin studying the night sky, it is hard to resist the urge to rush out and buy a telescope. But, you will do well to proceed slowly. You need to evaluate many personal factors before deciding on a particular telescope.
If you don't already own a pair, binoculars are a wise first choice in astronomical equipment. Binoculars are quite useful in learning the sky, due in part to their wide field of view. Additionally, the view through binoculars is properly oriented (right side up) making it easy to see where you are pointed. Binoculars are relatively inexpensive, offer respectable performance, are highly portable, and are easy to use - all great reasons to consider obtaining a pair.
The purpose of any telescope is to collect light and bring it to a point of focus so that it may be magnified and studied through an eyepiece. Each of the three designs briefly discussed above accomplish this differently; but each design performs satisfactorily, and each possesses their own special values.
Selecting a particular telescope depends on your individual needs and desires. Parameters to be considered include, but are not limited to, cost, portability, versatility, usability and appearance. Also worthy of consideration are your plans for using the telescope both now and in the future. Many amateur astronomers own more than one telescope in an effort to satisfy their varied interests.
Some amateur astronomers build their own telescopes - mostly large aperture Newtonian reflectors on Dobsonian mounts.
What follows is a general outline for choosing the right telescope.
First, determine your budget for this purpose. At this stage it is good to provide yourself a range within which you can work. Remember, whatever telescope you choose will also require the purchase of some accessories, so allow room for this in your budget projections. Just keep in mind that with telescopes, like everything else, you get what you pay for.
The first point of consideration is what purpose will the telescope serve. What type of observing are you interested in? Do you like viewing the moon and planets? Or perhaps you are enamored with distant galaxies. Is astrophotography a potential interest somewhere along your journey? Each type of telescope offers its own strengths and weaknesses.
For observing the moon and planets, you should be most concerned with image sharpness. The moon and planets are quite bright, so you needn't be too concerned with gathering more light. In this case a refractor may be the best choice. An achromatic refractor will show some false color along the edges of brighter objects. This phenomenon, known as chromatic aberration, can be eliminated by choosing the more expensive apochromatic refractor. It can also be greatly reduced by using the proper filter on an achromatic design. A refractor to be used for planetary work should have a focal ratio no smaller than F/8; faster refractors don't provide quite enough magnification.
Most refractors will prove to be too small (in aperture) for all but the very brightest deep sky objects. For this observational tract the reflector is best suited. This is because here we are primarily interested with light gathering ability and image brightness. Reflectors offer the most value per inch of aperture of all telescope designs. So, it would seem that bigger is better - so one should buy the largest aperture one can afford, right? Mostly, this is true... but don't forget to factor in portability. Most folks would do well to choose either a 6" or 8" reflector as a first telescope.
These sizes offer decent light gathering ability while still remaining quite portable. Of course, this (like most decisions) will depend on personal considerations and preferences. Just one word of caution: don't select a telescope whose size will limit its use. Sure, the views through a 17½" reflector from a dark site are awesome - but are you willing to lug around a telescope tipping the scales near 200 pounds? For those whose interests lie in deep space, large apertures are a necessity. To provide large aperture while maintaining some semblance of portability, a unique design has emerged: the truss-tube dob. This telescope is a Newtonian reflector on a Dobsonian (alt-az) mount... with the added ability of being able to be quickly and easily broken down into manageable components.
What if your particular situation dictates that you obtain only the smallest of telescopes, due to storage or portability concerns? A long focal-ratio refractor or a reflector may be too big and cumbersome. One potential candidate is the short-tube refractor. The most common examples here have objective lenses ranging from 70 mm to 120 mm in diameter. You may also consider the catadioptrics here, but they will be considerably more expensive than the short-tube, short-focus refractors.
For many new amateur astronomers the catadioptric design offers the greatest compromise. They are extremely versatile, lending themselves well to the study of the moon and planets, double stars, or deep sky objects. This versatility, combined with their available modern electronics, makes them a popular choice. Additionally, most SCTs are available as a package that includes the telescope, mount, tripod... maybe even an eyepiece or two. If your budget can accommodate their cost (between $1,000 and well, let's leave an upper number out), the catadioptric telescope can offer a lifetime of observational enjoyment and satisfaction.
To ensure that you make the best possible decision, you need to do your homework. Research all types of telescopes. Look through as many different kinds as possible. Ask lots of questions. Visit a dealer and carefully inspect the products. Ask lots of questions. Visit the library and lots of websites. Read. Alot. Ask lots of questions. Try to determine where your interests lie. Read some more.
Its been said before, but it bears repeating: the best way to select the proper telescope is to avail yourself of the company of others who share your interests. The best way to accomplish this is to join a local astronomy club!
Where To Buy a Telescope
First of all, avoid buying a telescope in a department store, a toy store or from one of those cable-TV shopping programs. Steer clear of any telescope that is marketed by its magnification... disappointment will surely follow.
If you are fortunate enough to have a local telescope dealer, by all means support them! Even if you have to spend a few extra dollars, they usually offer decent pricing, a showroom where you can actually see some of the products, and - by far the biggest advantage - great support. Dealers can answer most questions, and some can either perform or facilitate repairs.
Local dealers:
Another source of telescopes is, like most other things, the Internet. There are many dealers with an online presence. Some of the larger online retailers are listed below:
Also recommended is to use your favorite search engine to find information regarding a particular manufacturer. Most telescope makers maintain a website replete with technical specifications.
Another avenue to pursue for astronomical equipment is the second-hand, previously-owned, used market. Most amateurs take really good care of their equipment, so it isn't unusual to find a real bargain going this route. You can check the classifieds in your local newspaper, but there usually aren't many items even listed there.
Another source is from within your astronomy club. Post a 'wanted' ad in the newsletter, or send a 'wanted' notice out on the listserver.
Probably the best source for used astro goodies is Astromart. You really have to check out this website!
Which Binoculars To Buy
For the price of a mediocre-quality, inexpensive first telescope you can obtain a good pair of binoculars. This would be a wise decision.
The first criteria involves the type of prisms the binoculars use: roof prisms or porro prisms. Generally speaking, the views through porro prisms will be both brighter and sharper. You will discover that porro prisms are typically made from two types of glass: BK-7 (borosilicate) or BaK-4 (barium crown). Because BaK-4 prisms pass all of the light that enters them, they will produce sharper images.
As you would expect, binoculars using BaK-4 glass will cost a little more than BK-7 glass. Avoid gimmicks such as ruby-colored lenses.
While perusing binoculars, you will notice that they are specified by two numbers (7x35 or 10x50, for example). The first number is the magnification provided, and the second number is the diameter (in millimeters) of the two objective (front) lenses. In order to reach a decision regarding magnification, you must ask yourself how you plan to support them. If you want to be able to hand-hold the binoculars, you should not go above 9x (power). For most folks, 10x binoculars are nearly impossible to hold steadily by hand for long periods of time.
Selecting the proper objective diameter involves a little more thought; bigger is not always better. To provide for the most enjoyable views, you need to try to match the beam of light exiting the binoculars eyepieces (the exit pupil) to the diameter of your pupil. The pupil diameter varies from person to person, ranging from about 2.5 mm in bright light to 7 mm in dark conditions. The exit pupil for any binoculars is calculated by dividing the objective diameter by the magnification. For example: 7x35 and 10x50 binoculars yield a 5 mm exit pupil, 10x70's yield 7 mm. A good rule of thumb is to choose a pair that yields an exit pupil in the 4 to 5 mm range. They will be comfortable for use in the suburbs or at a dark site.
Another useful bit of information is the rated field of view. This value is usually noted on the back of the binoculars, near the eyepieces. You may see something like '272 feet at 1,000 yards'. What does that mean? Simply stated, it means that an object 272 feet wide and 1,000 yards away will just fit into the field of view. For astronomical usage, you can convert this value to something more useful - the angular field of view - by dividing the 'feet' by 52.5. Using our example: 272 ÷ 52.5 = 5.18, or just over 5° of sky. Some binoculars will have the field of view stated in degrees already.
If you have decided to investigate binoculars over 10x, you will also need to investigate a mount. This can be as simple as a sturdy photo tripod (with an 'L' bracket to attach the binoculars to the tripod) or as elaborate as a sophisticated parallelogram mount.
What Is a Reflector?
Reflecting telescopes use mirrors to gather light; mirrors reflect the light. Newtonian telescopes (also known as catoptrics) usually use a concave parabolic primary mirror (longer focal ratios may use spherical primaries) to collect and focus incoming light onto a flat secondary (diagonal) mirror. This secondary mirror reflects the image out of an opening at the side of the main tube and into the eyepiece. The Newtonian reflector is named after its inventor, Sir Isaac Newton.
Advantages
-
Lowest cost per inch of aperture.
-
Reasonably compact and portable up to focal lengths of 1,000 mm.
-
Excellent for deep sky observing due to the generally fast focal ratios (f/4 to f/8)
-
Reasonably good for lunar and planetary work.
-
Good for deep sky astrophotography, though not as convenient as a catadioptric in this regard.
-
Low in optical aberrations.
-
Nice, bright images.
Disadvantages
-
Open optical tube design allows image degrading air currents and contaminants that can damage the mirror coatings over time.
-
Require a little more maintenance than other designs, such as routine collimation.
-
May suffer from off-axis coma.
-
Apertures over 10" can be heavy, bulky and expensive.
-
Generally not suited for terrestrial observations.
-
Slight light loss due to secondary mirror obstruction, as compared to refractors.
Did you ever wonder why most research telescopes are reflectors? Imagine searching for a small item in a large, dark room. How will you find it? What would make your search easier: a magnifying glass or a flashlight?
The Universe is vast and the light coming from other stars and galaxies is very dim. For example, the nearest large galaxy to our own, Andromeda, is about six times the apparent diameter of the Moon, but the Moon seems much larger than Andromeda even through binoculars. Just enlarging the sky won't solve the problem -- you don't just need a magnifying glass to find something small in the darkness. You need more light, so you need to make a telescope that will collect a lot of light. The larger the telescope's diameter, the more light you can collect.
But, you also need to have some way of focusing all the light you collect. Galileo's idea of using glass lenses to focus the light from stars was thought to be the best way to do this for some time. But, one problem with glass lenses is that, like prisms, they don't bend light of all wavelengths evenly. So, even if you make a lens very carefully, when you take a picture of a star with that single lens, you get a blob that is bluer on one edge and redder on the other edge. This phenomenon is called chromatic aberration. You can minimize this effect by using lenses made from two kinds of glass, crown and flint.
You can also use special filters to let in only one color of light -- then all the light is aberrated in the same way (since it's monochromatic). Fortunately, mirrors reflect light of all (visible) wavelengths in exactly the same way, so if you have a reflecting telescope, you don't have to worry about chromatic aberration.
What Is a Refractor?
Refracting telescopes use lenses to gather light; lenses refract, or bend, the light. Also known as a dioptric, refractor telescopes are what most folks picture when they hear the word 'telescope'. Refractors have a long, thin tube through which light passes in a straight line from the front objective lens directly to the eyepiece, at the opposite end of the tube.
While investigating the refractor market, you may notice what appears to be two rather distinct classifications, relative to price. Refractors are available in two types: the standard achromatic instrument and the more expensive apochromatic design. The difference in these instruments is all about the glass: the composition of the objective lenses; the number of elements, coatings and the type of glass used to make the lenses. These design differences are necessary to combat a problem inherent in the refractor design: chromatic aberration, which is visible at the eyepiece as the false-color fringes at the edges of bright objects.
A typical achromatic telescope uses an objective comprised of two elements, a doublet. The Celestron C102, Meade AR-5 or 6, Vixen 102M and Stellarvue AT1010 are examples of this design. In fact, most refractors sold fit into this category, with most costing considerably less than $1,000.
The next step up in optical quality is the result of replacing one of the two objective elements with extra low dispersion glass. The resultant telescope is referred to as an ED achromat. The TeleVue Ranger or Pronto and the Vixen ED80S are examples of this level of optical quality.
The next level, the apochromat, uses three elements in the objective lens, a triplet design. Additionally, one or more of the elements may be made from an exotic glass such as fluorite. Examples in this category include most of the offerings from Astro-Physics, TeleVue or Takahashi.
Advantages
-
Easy to use and reliable due to the simplicity of design.
-
Little or no maintenance required.
-
Excellent for lunar, planetary and binary star observing especially in larger apertures.
-
Good for distant terrestrial viewing.
-
High contrast images with no secondary mirror or diagonal obstruction.
-
Color correction is good in achromatic designs and excellent in apochromatic, fluorite, and ED designs.
-
Sealed optical tube reduces image degrading air currents and protects optics.
-
Objective lens is permanently mounted and aligned.
Disadvantages
-
More expensive per inch of aperture than reflectors or catadioptrics.
-
Heavier, longer and bulkier than equivalent aperture reflectors and catadioptrics.
-
The cost and bulk factors limit the practical useful maximum size objective to small apertures.
-
Less suited for viewing small and faint deep sky objects such as distant galaxies and nebulae because of practical aperture limitations.
-
Focal ratios are usually long (f/11 or slower) making photography of deep sky objects more difficult.
-
Some color aberration in achromatic designs (doublet).
-
Poor reputation due to low quality imported toy telescopes; a reputation unjustified when dealing with a quality refractor from a reputable manufacturer.
Good Telescope Mounts
In a word: stability. For the most part, a telescope is only as good as its mount. The world's best optics can be rendered useless if not properly supported. A telescope magnifies everything, including vibrations. Additionally, the adjustment controls should operate smoothly and precisely - they'll be used to allow for tracking of the slow and apparent movement of the stars. Motor drives are a wonderful addition visually, and an absolute necessity photographically.
Basically, the job of the telescope mount is twofold:
-
To support the telescope firmly, allowing for visual or photographic usage uninterrupted by image movement.
-
To provide a system to accurately guide and point the telescope.
All mounts fall into one of two basic mount types: Alt-Azimuth or Equatorial.
Alt-Azimuth
This is the simplest design, incorporating two basic movements: azimuth (side-to-side or horizontal motion) and altitude (up-and-down or vertical motion). A decent mount of this type should employ some kind of slow-motion controls, to facilitate smooth tracking motion. An additional component, an equatorial platform, can be utilized to compensate for this mounts tracking concerns.
Equatorial
This is a much more sophisticated design than an alt-az mount. It is superior for both visual and photographic applications. As the earth rotates around its axis, the stars appear to move across the sky. When using a telescope on an alt-az mount, the stars will quickly (depending on magnification) drift out of the field of view along both axes. This requires a sort of 'stair-stepping' manual tracking method, somewhat of an acquired skill. However, when using an equatorially mounted telescope, the tracking motions are smoothly and easily controlled using the slow-motion knobs, or automatically through the addition of drive motors.
The equatorial mount rotates on one axis (the polar or right ascension axis). This axis is adjusted to the latitude of the observing location and aligned to make it parallel to the Earth's axis. If this axis is turned at the same apparent speed as the Earth, but in the opposite direction, the objects in the eyepiece will appear to remain motionless. This is the function of a clock drive.
Most modern catadioptric instruments utilize another type of mount: the fork mount. It is basically an alt-az mount with electronics to control tracking. The more sophisticated models feature full go-to functionality, offering 'point-and-shoot' astronomy. A wedge can be added to transform the alt-az fork mount into an equatorially mounted fork, most useful for photographic applications.
Catadioptric Telescopes
Catadioptric systems are those which make use of both lenses and mirrors for image formation. This contrasts with catoptric systems (reflectors) which use only mirrors and dioptric systems (refractors) which use only lenses.
The most common variety of 'cats', as they affectionately referred, are the Schmidt-Cassegrains. They come in many sizes, but the 8" variety is by far the most popular, offering a nice balance between light-gathering ability and portability. The Schmidt-Cassegrain telescope is also referred to as SCT.
While the Schmidt-Cassegrain design is by far the most popular catadioptric, other types worth mentioning include:
-
Maksutov-Cassegrain - uses a meniscus lens to supply correction to the spherical mirror system, instead of the SCT's corrector plate. This design practically eliminates the need for collimation.
-
Schmidt-Newtonian - utilizes a Schmidt lens as a corrector for the main mirror, support for the secondary mirror, and as a seal for the optical tube. This design excels at low powers.
-
Maksutov-Newtonian - uses a Maksutov meniscus lens for correction.
Cats provide good views of al but the faintest objects. They are quite compact and fairly easy to use. By far, the widest variety of accessories are available for this breed of telescope. Most manufacturers offer this type of telescope on a fork-mount, usually with go-to computerization and GPS capabilities. Cats are also available in OTA form - suitable for equatorial mounting. Their major drawback is that they can be relatively expensive when compared to reflectors of similar aperture.
Advantages
-
Best all-purpose design, combining the optical advantages of both lenses and mirrors.
-
Excellent optics providing sharp images over a relatively wide field.
-
Excellent platform for deep sky observing or astrophotography.
-
Closed-tube design reduces image degrading air currents.
-
Very compact and portable.
-
Easy to use.
-
Durable and virtually maintenance free.
-
Most versatile telescope design.
-
More available accessories than any other design (well, this may be a disadvantage too!)
Disadvantages
-
More expensive than reflectors of equal aperture.
-
Doesn't fit the pre-conceived image of a telescope.
-
Slight light loss due to secondary mirror obstruction, in comparison to refractors.
The first compound telescope was made by German astronomer Bernhard Schmidt in 1930. The Schmidt telescope had a primary mirror at the back of the telescope, and a glass corrector plate at the front of the telescope to remove spherical aberration. The telescope was used primarily for photography, because it had no secondary mirror or eyepieces -- instead, photographic film was placed at the prime focus of the primary mirror. Today, the Schmidt-Cassegrain design, which was invented in the 1960s, is the most popular type of telescope; it uses a secondary mirror that bounces light through a hole in the primary mirror to an eyepiece.
The second type of compound telescope was invented by a Russian astronomer, D. Maksutov, although a Dutch astronomer, A. Bouwers, came up with a similar design in 1941, before Maksutov. The Maksutov telescope is similar to the Schmidt design, but uses a more spherical corrector lens. The Maksutov-Cassegrain design is similar to the Schmidt Cassegrain design.
Eclipses
An eclipse is an astronomical event that occurs when an astronomical object or spacecraft is temporarily obscured, by passing into the shadow of another body or by having another body pass between it and the viewer. The term eclipse is most often used to describe either a solar eclipse, when the Moon's shadow crosses the Earth's surface, or a lunar eclipse, when the Moon moves into the Earth's shadow. For more info see: A Student's Guide to Solar and Lunar Eclipses